QVLS-Q1: Der Quantencomputer aus Niedersachsen
Unser Ziel ist es, bis Ende 2025 eine 50 Qubit-Maschine auf der Basis von Ionenfallen zu realisieren. Diese Technologie wird derzeit als einer der weltweit vielversprechendsten Ansätze für skalierbare Quantencomputer angesehen, da dieser einen Betrieb bei Raumtemperatur und mit geringsten Fehlerraten ermöglicht. Für den Bau von QVLS-Q1 wird die in Niedersachsen etablierte Weltspitzen-Expertise mit Ingenieuren und der Industrie gebündelt und somit die gesamte Kette für den Bau eines Quantencomputers umfasst. Die Zusammenführung aller erforderlichen Expertise unter einem Dach – von der Nanotechnologie bis zu Quanten-Algorithmen oder der Herstellung von Ionenfallen-Chips – ist ein überzeugendes Alleinstellungsmerkmal des ambitionierten Projekts sogar im weltweiten Vergleich.
Hinweis: Nach der Aktivierung des Videos werden Daten an den jeweiligen Anbieter übermittelt. Weitere Informationen finden Sie in unserer Datenschutzerklärung.
Video abspielen
QVLS-Q1 Teams
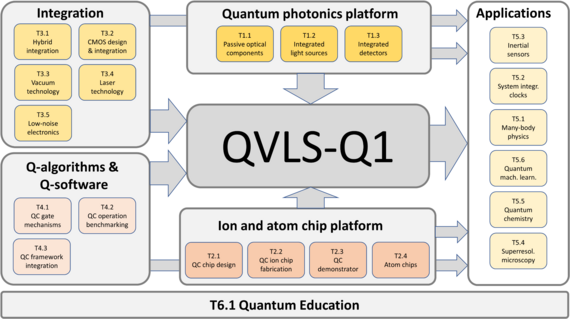
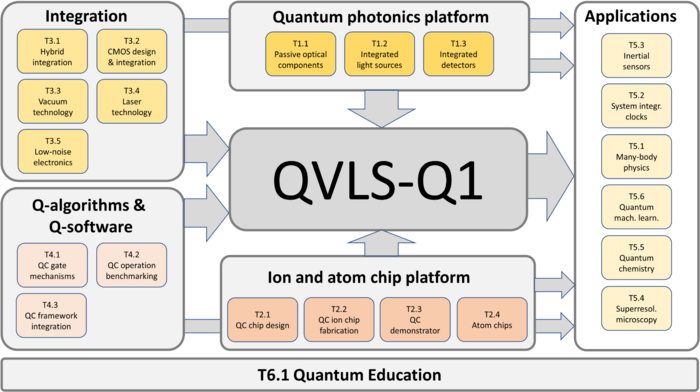
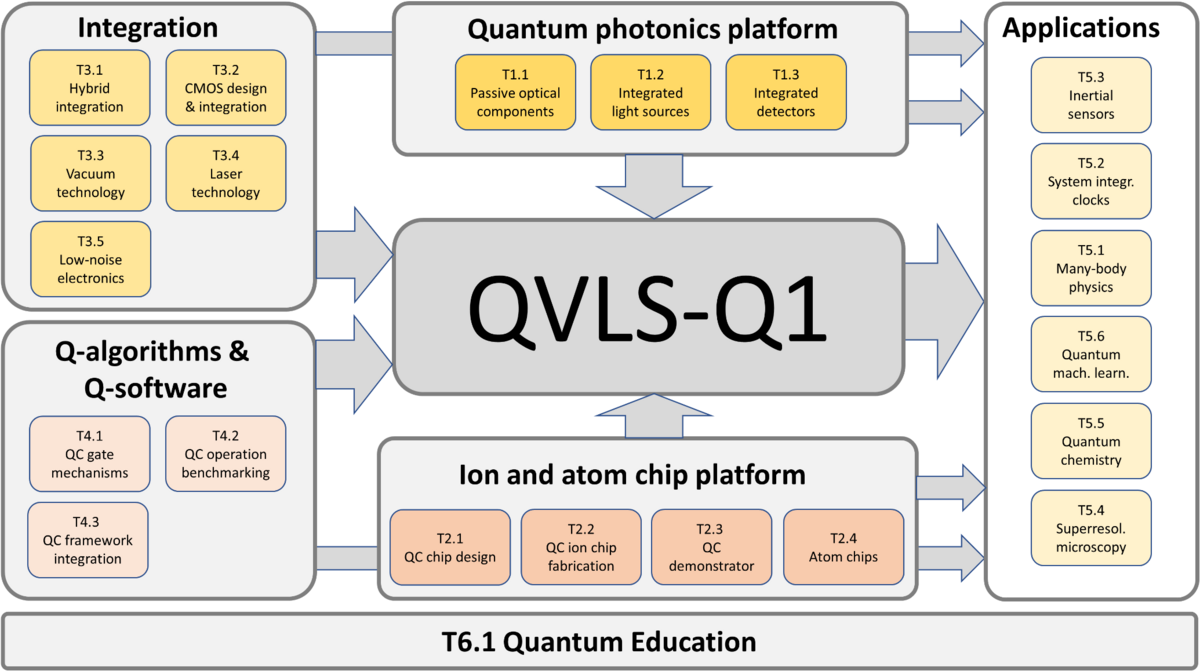
Überblick der verschiedenen Plattformen und Teams (Tx.y), welche zu QVLS-Q1 und Zielanwendungen beitragen.
-
T1: The quantum photonics platform
The quantum photonics platform requires the development of a highly integrated optical interposers with waveguides, optical switching elements and phase control, active laser sources in the blue/UV spectral range with required beam quality, as well as single photon detectors integrated with light guiding and light emitting components.
- T1.1 Passive optical components in 3D, optical integration, optical interfaces, waveguides (Kroker, Mehlstäubler)
- T1.2 Integrated light sources and active optical elements (Hartmann, Waag)
- T1.3 Integrated single photon detectors (SPAD, SNSPD) (Waag, Schilling, Raupach, Bieler)
-
T2: The ion and atom trap platform
The ion and atom trap platform will be based on a design team entirely devoted to simulation and chip design, complemented by teams working on the assembly of the quantum computer, developing process technology for the ion trap chip, and for developing chips for atom interferometry.
- T2.1 Surface ion-trap chip design (C. Ospelkaus, Schöbel)
- T2.2 Process technology for surface-trap fabrication (Bautista, C. Ospelkaus)
- T2.3 Ion-trap quantum computer demonstrator assembly (C. Ospelkaus, Schmidt)
- T2.4 Multi-functional atom chips (Rasel, Mehlstäubler, Wurz, Kroker)
-
T3: Hybrid integration process development
The integration activities include teams working on concepts for hybrid integration, CMOS design, vacuum and laser technology and low noise electronics with the goal to miniaturize the technological environment for operating the quantum computer.
- T3.1 Hybrid integration process development (Wurz, Bautista, Waag, Schilling, Kroker, Mehlstäubler)
- T3.2 CMOS-Design and CMOS Integration (Issakov, Waag, C. Ospelkaus)
- T3.3 Advanced vacuum technology (Schmidt, Wurz, Lisdat, Schilling)
- T3.4 Laser technology and breadboarding (Schmidt, C. Ospelkaus, C. Lisdat)
- T3.5 Ultra-low-noise control electronics (Schilling, Schmidt, Manteuffel, Schöbel)
-
T4: Quantum algorithms and quantum software
Quantum algorithms and quantum software will be developed by teams working on gate mechanisms, the quantum computing interface to external software environment, automation of the operation of a quantum computer and on quantum machine learning.
- T4.1 Gate mechanisms and quantum optics (Hammerer, Werner)
- T4.2 Automation, benchmarking and operation of ion-trap quantum computer (C. Ospelkaus, Schmidt)
- T4.3 QC interface / QISKIT integration / cloud access (Weimer)
-
T5: Applications
An additional group of teams focuses on direct applications of the developed quantum processor, such as in quantum machine learning, quantum simulations and quantum chemistry, and on particularly mature spin-off technology used for addressing applications in specific fields such as transportable clocks, compact inertial quantum sensors and super-resolution microscopy. These applications offer additional exploitation channels for enabling technologies developed on the way, hence increasing the technological and scientific impact as well as the added value even beyond quantum computation.
- T5.1 Many-body applications / digital quantum simulations (Santos, Karrasch, Recher, Osborne, Weimer, Werner)
- T5.2 System integration for high-performance transportable optical clocks (Lisdat, Schmidt)
- T5.3 Compact inertial quantum sensors (Rasel, Wurz, Schubert)
- T5.4 Superresolution microscopy (Waag, Lalkens, Dammeyer)
- T5.5 Quantum chemistry (S. Ospelkaus, Schmidt)
- T5.6 Quantum machine learning (Osborne, Anand, Heurs)
-
T6 Quantum Education
In order to establish an ecosystem of skilled quantum workforce we will develop educational resources tailored to the needs raised by the advance of quantum computers. This includes the development of innovative concepts, materials and curricula for physics and engineering students as well as training programs for the industry workforce.
Core Team: Rainer Müller, Tobias Voss, Nicolas Spethmann